Microbiology
New and Improved! A supercharged antibiotic to fight superbugs
New antibiotics are needed to treat infections caused by bacteria that can no longer be killed by current drugs. We have improved an existing antibiotic by chemically adding on extra pieces that make it bind more strongly to bacteria.
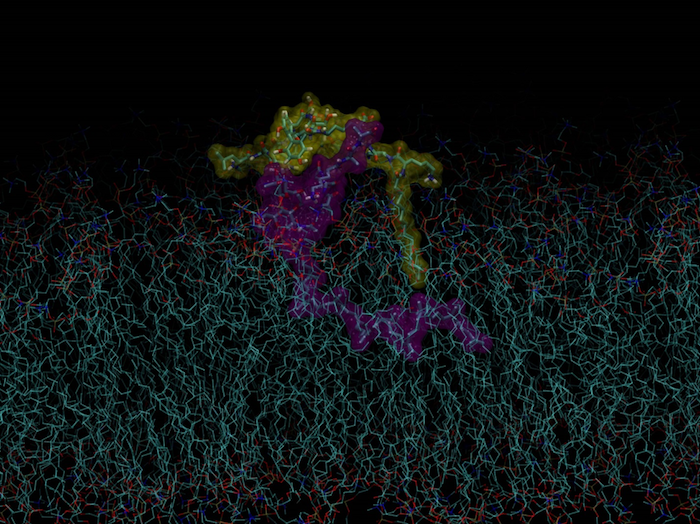
Have you taken an antibiotic lately? You're not alone. Almost one in two people will have been dosed with an antibiotic over the last year. Unfortunately, all this antibiotic use is causing the bacteria to become more and more resistant to the drug's effects. We are now facing a future where antibiotics may no longer work, and bacterial infections become much more deadly. This problem is made worse because new and improved antibiotics are becoming rare. Our recent publication, with the not-so-catchy title of, "Protein-inspired antibiotics active against vancomycin- and daptomycin-resistant bacteria", describes one approach we're taking to try to help solve the antibiotic crisis. We'll explain below exactly what that title means.
Our strategy is based on trying to develop a drug that targets pathogens (harmful bacteria) in a fundamentally different way. Almost all drugs nowadays interact with one of two elements that are essential for life: proteins or DNA/RNA. However, there is a third component that is generally overlooked - the membranes that encase living cells. Membranes coat not only our (mammalian) cells, but also the bacterial cells that infect us. While human and bacterial cell membranes have generally similar structures, they also have some very important differences. Nature already uses these differences to direct where some molecules end up. Could we use the same approach to develop a drug that targets only bacterial cell membranes? (This is the "protein-inspired" part of our article title). By doing this, we might be able to reduce some of the detrimental side effects that result when a drug cannot distinguish between different cell types, instead interacting with all of them.
We decided to test whether membrane targeting would work with antibiotics, as with this class of drugs we could take advantage of the more substantial differences between human cells and bacterial membranes. Different types of antibiotics work in different ways - some prevent the bacteria from making new proteins by acting on the synthetic machinery inside the bacteria, while others interfere with construction of the cell wall that holds the bacteria together, or create holes in the membrane so that the cell contents leak out.
Of all the potential antibiotics available, we chose vancomycin as the core drug to modify to selectively target it to bacterial membranes. Vancomycin is still an important antibiotic for treating infections, but resistance is increasing, making it lose effectiveness. It kills bacteria by binding to a building block of the bacterial cell wall, preventing the cell wall from being constructed. Bacteria under attack by vancomycin are unable to grow or reproduce, because for both growth and reproduction, more cell wall would have to be produced. Importantly for our strategy, this cell wall building block is found just under the cell wall, where it is embedded into the next layer encasing the bacterium, the cell membrane. By targeting vancomycin to the bacterial membrane, we funnel it right to where it's needed.
The cell membrane itself is made up of many types of molecules, but the main component is lipids, which have a fatty tail and non-fatty head, some with a negative charge (like the negative end of a battery). Vancomycin is able to be chemically modified, so we can attach to it a membrane-targeting substituent without disturbing its usual antibacterial activity. We joined together three different components that could be adjusted independently, like putting together different colour combinations of three Lego blocks. These components consisted of (1) a "linker", which joined the membrane-targeting substituent and vancomycin, (2) an "electrostatic effector peptide segment (EEPS)" that is attracted to the bacterial membrane, and (3) a fatty component designed to interact with the fatty tail of the membrane lipids. We named these modified versions of vancomycin the "vancapticins".The EEPS is the "protein-inspired" part of the molecule, and just like the positive side of one magnet is attracted to the negative side of another one, we used a positive charge on the EEPS to bind to the negative charge on the bacterial lipids.
We then made hundreds of new versions of vancapticins by making small changes in the molecule. Some of these new versions were more than 10 times more effective than vancomycin at killing bacteria! We soon found that the size of the EEPS could be greatly reduced, which simplified the new structure and boosted the potency another 10-fold. Then, the final breakthrough came when we changed the original linker (component "1" above), to a linker that living organisms could not destroy.
With all these changes, there were still several promising vancapticin candidates. We ran them through a series of tests to compare how effective they were at killing bacteria without causing harm to non-bacterial cells. Other tests showed that bacteria don't quickly develop resistance against these new drugs. Finally, and most importantly, we proved that the new vancapticins cured several types of bacterial infections in mice with much greater effectiveness than vancomycin. Unlike mouse models of cancer that often do not predict the success of human testing, these infection models usually foretell whether antibiotics will work in humans.
We have continued to work on developing improved versions of the vancapticins, and hope that they will help address the looming threat of antimicrobial resistance. The biggest barrier to continuing their development is finding a viable commercial route, as there is little money to be made in selling antibiotics, especially when compared to other types of drugs. This project has involved many people across numerous institutions for more than a decade. Our main motivation, like the antibiotic pathfinders in the 1940s, has been to help prevent people from dying from bacterial infections.
Original Article:
M. A. T. Blaskovich et al., Protein-inspired antibiotics active against vancomycin- and daptomycin-resistant bacteria. Nat Commun 9, 22 (2018)Next read: Vaccine hope against a sexually transmitted disease: the answer to the burgeoning rise in a superbug by Helen Petousis-Harris
Edited by:
Dr. Carlos Javier Rivera-Rivera , Managing Editor
We thought you might like
Fighting back antibiotic resistance: a new hope from the soil
Feb 24, 2016 in Microbiology | 4 min read by Dan KramerCollateral damage: antibiotics disrupt the balance in the gut
Jun 2, 2016 in Microbiology | 3.5 min read by Katri KorpelaInvisible allies for healthy juvenile growth
Oct 12, 2016 in Microbiology | 4 min read by Martin SchwarzerRed in Tooth and Claw: another weapon against antibiotic resistance
Oct 3, 2017 in Microbiology | 3.5 min read by Nicholas A. IsleyMore from Microbiology
Monoclonal antibodies that are effective against all COVID-19 -related viruses
Jan 31, 2024 in Microbiology | 3.5 min read by Wan Ni ChiaPlagued for millennia: The complex transmission and ecology of prehistoric Yersinia pestis
Jul 31, 2023 in Microbiology | 3 min read by Aida Andrades Valtueña , Gunnar U. Neumann , Alexander HerbigHow cellular transport can be explained with a flip book
Jun 5, 2023 in Microbiology | 3 min read by Christina ElsnerThe Achilles’ heel of superbugs that survive salty dry conditions
Apr 24, 2023 in Microbiology | 4 min read by Heng Keat TamNew chemistry in unusual bacteria displays drug-like activity
Mar 21, 2023 in Microbiology | 3.5 min read by Grace Dekoker , Joshua BlodgettEditor's picks
Trending now
Popular topics