Maths, Physics & Chemistry
How lab-grown blood vessels can help us understand malaria
Our body has a vast amount of small blood vessels that deliver and take away wastes. These small blood vessels can clog in many diseases including malaria, causing tissue damage and organ failure. We report a breakthrough in developing a lab-grown model of the tiniest blood vessels. This model will help study different diseases, and seek better treatment options for clogged microcirculation.
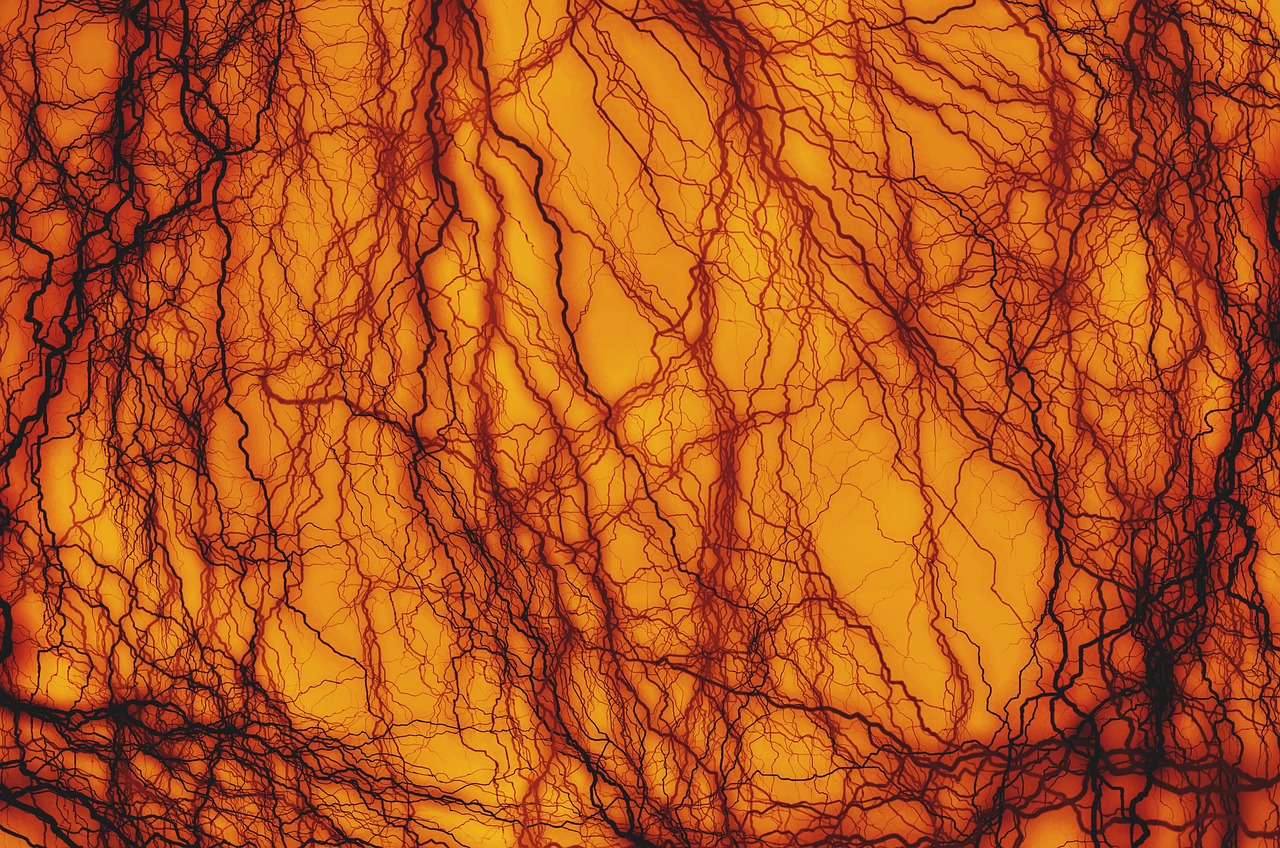
Blood circulates a hundred thousand times in our body each day to provide nutrients and support to tissues. This circulation is carried out by blood vessels in a highly organized and efficient transport system. Large vessels are somewhat like highways, carrying large volumes of blood from the heart to distant organs. Within each organ, blood is distributed along a network of much smaller blood vessels called the microcirculation, which can be 20 times smaller than a human hair in diameter. During this journey, red blood cells carry oxygen to all the tissues in the body. Red blood cells have been designed by nature to literally squeeze through the microcirculatory network and can even pass through openings smaller than their circumference. Under normal circumstances, red blood cells pass smoothly through the circulation. Specific diseases, such as sickle cell trait and malaria, alter red blood cells and make them less deformable and sticky, so they clog the microcirculation, like a traffic jam. This obstructed blood flow will cause damage to tissues and organs, and in the worst cases, death. It has been recognized for over one hundred years that people dying from P. falciparum malaria had clogged microvessels. However, studying this process is challenging outside of human infections. Recently, scientists have grown blood vessels that are made from human endothelial cells in the lab to mimic those in humans. The critical challenge has been developing a way to grow these vessels as small as they are in the human body. We reported a breakthrough in developing a lab-grown model of the tiniest blood vessels. This model will help screen and study different varieties of diseases and seek better treatment options for clogged microcirculation.
Malaria is a life-threatening disease that affects more than 200 million people worldwide yearly. The deadliest form of the disease is caused by the parasite Plasmodium falciparum, which is transmitted into humans through mosquito bites. In the blood stage of infection, parasites grow and multiply within red blood cells. Once a parasite is inside of a red blood cell, it begins to remodel the red cell membrane causing the display of knob-like protrusions and adhesive proteins on the surface. These changes make the cells less deformable and allow them to adhere to the endothelial cells lining the inside of blood vessels, but what factors cause vessel-clogging? This has been a challenging question for scientists to study as telling what is occurring in the smallest blood vessels in patients is nearly impossible with current technologies.
To overcome these limitations, we set out to develop a lab-grown model of a human capillary made from human endothelial cells. To do this, we employed a technique known as soft-lithography in which we can "stamp"; patterns of channels into malleable gels. By flowing endothelial cells into prefabricated channels, the cells attach to the walls and over a few days form completely enclosed endothelial tubes, mimicking our blood vessels. The challenge we faced was to create stable blood vessels that were as small as human capillaries, which can be as small as 5 micrometers or 1/200th of a millimeter in diameter. To do this, we used a specialized laser capable of creating extremely tiny channels for endothelial cells to grow into.
The question our study addressed is whether malaria-infected red blood cells got stuck in the microcirculation because the parasite was so large that it prevented the red blood cell from squeezing through or if the parasite adhesion proteins were the problem. First, we showed that healthy red blood cells are easily squeezed through the narrow vessel spaces without clogging, much like what occurs in humans. By comparison, as malaria-infected red blood cells traveled through the capillaries, they became stuck in the channels, leading to similar clogging as what is seen in malaria patients. However, if we used a protease to remove the parasite adhesion proteins from the surface of the red blood cells, then they no longer got trapped. Thus, our model can replicate the microvessel clogging that occurs in human infections, and our findings suggest that interventions will need to target the parasite adhesion proteins to unblock clogged vessels.
We hope that continuing to study these traits could lead to improvements in the development of targeted drugs, which could help to reduce the mortality rate of malaria. Additionally, our future work will be to continue to improve the in vitro capillary system to use for studying other blood and vascular diseases.
Original Article:
Arakawa C, Gunnarsson C, Howard C et al. Biophysical and biomolecular interactions of malaria-infected erythrocytes in engineered human capillaries. Sci Adv. 2020;6(3):eaay7243.
Next read: The Trojan mosquito: an in-house parasite defends against malaria by Enock Mukibetti Mararo , Lilian Mbaisi , Edward Makhulu Edmond
Edited by:
Massimo Caine , Founder and Director
We thought you might like
Driving down malaria
Jul 18, 2017 in Health & Physiology | 4 min read by Andrew Hammond , Xenia Karlsson , Ziyin WangA special delivery for malaria-transmitting mosquitoes
Dec 18, 2019 in Health & Physiology | 3.5 min read by Etienne Bilgo , Brian LovettMore from Maths, Physics & Chemistry
Testing gravity through the distortion of time
Sep 20, 2024 in Maths, Physics & Chemistry | 3 min read by Sveva CastelloStacking molecular chips in multiple dimensions
Aug 30, 2024 in Maths, Physics & Chemistry | 3 min read by Lucía Gallego , Romain Jamagne , Michel RickhausReversible Anticoagulants: Inspired by Nature, Designed for Safety
Jun 12, 2024 in Maths, Physics & Chemistry | 4 min read by Millicent Dockerill , Nicolas WinssingerDistance-preserving moves always keep a point fixed
May 18, 2024 in Maths, Physics & Chemistry | 4 min read by Shaula FiorelliA resonance triggers chemical reactions between the coldest molecules
Apr 5, 2024 in Maths, Physics & Chemistry | 3 min read by Juliana Park , Wonyl ChoiEditor's picks
Trending now
Popular topics