Health & Physiology
Forced to react: 3D printing can stretch a single cell
A cell is highly interactive and constantly exchanges ‘physical’ information with its surroundings. By combining a special 3D printing technique with smart materials, we developed micrometer-sized scaffolds to stretch single cells. This new approach helps us to better understand how individual cells sense and respond to external mechanical stimuli.
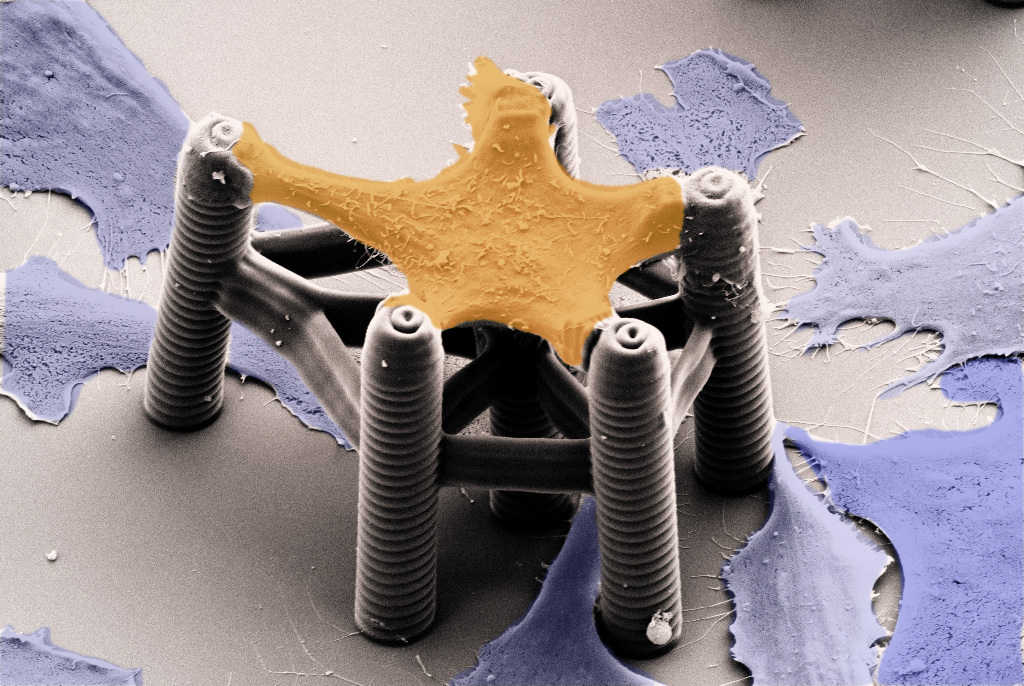
A cell is a tiny compartment, the most fundamental architectural unit of life. Like in the context of a whole body, every cell is highly interactive with its surroundings. As a result, cells gather and form functional communities such as tissues and organs.
In those communities, cells are not always tightly associated with each other but form a network with gaps. This extracellular space – filled with many key biomolecules – serves as a platform to exchange various information with cells. Traditionally, scientists have considered that essential cellular events – such as adhesion, migration, proliferation, and differentiation – respond to extracellular stimuli via biochemical reactions. However, recent studies increasingly suggest that the physical stimuli from the extracellular space can also control cellular events.
The field of cellular mechanobiology aims at understanding how cells respond to mechanical cues in their environment. While the body moves, tissues are stretched or compressed, triggering cellular reactions that are crucial for tissue maintenance or wound healing. Cells in these tissues react to such mechanical signals by generating intracellular forces of their own through interactions within the cytoskeleton – the dynamic network of protein filaments, extending throughout the cell's interior. The field of cellular mechanobiology aims at understanding how cells respond to mechanical cues in their environment.
It has been technically challenging to detect such intracellular forces as they are exceptionally weak. The detection methods thus usually require comprehensive calibrations and controls. These drawbacks complicate the simultaneous stimulation and detection of single-cell forces. As a result, the only currently available methods are either time-consuming or not applicable to cellular-scale.
To overcome these limitations, we developed 3D microstructures aimed at applying mechanical stress to single cells and analyzing their response. Cells – with a typical size of 20-60 micrometer – can only exert forces in the very small-scale (nanonewton range). We employed a printing method known as 3D laser lithography. This technique enabled the fabrication of tiny 3D scaffolds that hold a single cell and precisely guide its adhesion sites. In addition, these structures were sufficiently flexible to monitor the cell's small motion. By fine-tuning the structures, we could demonstrate cells in the scaffolds constantly pulling with different strengths.
However, such a "static" scaffold was not sufficient to simulate cell's reactions against a dynamic extracellular environment. To apply extracellular mechanical stimuli, we needed active structures that deform on demand. We achieved this by adding a class of materials called stimuli-responsive hydrogels to our scaffolds. Such "smart materials" can convert changes in their environment – including temperature, pH, and chemicals – into physical stimuli. Combining these hydrogels with the 3D laser lithography technique ultimately allowed us to fabricate single-cell-scaffolds that can reversibly control the cell's deformation on demand.
Next, we applied this single-cell-scaffold to study the response of cells to mechanical stretches. When stretched, cells actively counteracted and pulled in the opposite direction. After a while, they rearranged their intracellular forces and stabilized on a new force level. When the stretch was released again, cells relaxed and returned to their initial state. This indicates that cells possess a mechanical memory (also called mechanical homeostasis). Furthermore, we identified a component of the cytoskeleton, called NM2A, that plays a significant role in generating contraction forces and a mechanical memory. Cells lacking NM2A were no longer able to respond to external stimuli.
In conclusion, we introduced novel 3D printing-driven single-cell-scaffolds that can physically react to environmental stimuli. This new approach allowed us to learn how cells constantly adapt to the physical cues in their surroundings: cells can sense external mechanical stimuli and rapidly respond to them by reorganizing their intracellular structure and adjusting their internal force equilibrium. This ability is crucial for cells to maintain their integrity in a constantly changing physiological environment.
Our findings are only the tip of the iceberg, and our new approach has a great potential for answering important questions. Future studies should apply this technique to further our understanding of the molecular mechanisms that convert extracellular mechanical stimuli into intracellular biochemical reactions. Shedding light on the long-ignored physical aspect of cellular activities would give us clues to untangling complex cellular events.
Original Article:
Hippler, M. et al. Mechanical stimulation of single cells by reversible host-guest interactions in 3D microscaffolds. Sci. Adv. 6, (2020).
Next read: How platelets pull the strings by Ingmar Schoen , Sebastian Lickert , Viola Vogel
Edited by:
Dr. Akira Ohkubo , Associate Editor
We thought you might like
How to print a brain - the initial steps
Nov 8, 2016 in Maths, Physics & Chemistry | 3.5 min read by Nieves CuboHolographic sound fields shape 3D matter without a touch
Nov 15, 2023 in Maths, Physics & Chemistry | 4 min read by Kai MeldeWorld’s first microscale ‘transformer’ robot
Nov 26, 2020 in Maths, Physics & Chemistry | 2 min read by Tian-Yun Huang , Huiling DuanMore from Health & Physiology
Tobacco smoking and other exposures shut off cancer-fighting genes
Aug 31, 2024 in Health & Physiology | 3 min read by Jüri Reimand , Nina AdlerA hidden clock that times cytoplasmic divisions
Aug 30, 2024 in Health & Physiology | 3 min read by Cindy OwWhen two kinases go for a dance
Aug 2, 2024 in Health & Physiology | 4 min read by Ioannis Galdadas , Francesco Luigi Gervasio , Pauline JuyouxAwakening the thymus to cure SARS-CoV-2 infection: a matter of genes
Jul 27, 2024 in Health & Physiology | 3.5 min read by Stefano Marullo , Cheynier RemiKeeping the balance: How epigenetics monitors cancer genes
May 13, 2024 in Health & Physiology | 4 min read by Zach Gray , Madison Honer , Johnathan WhetstineEditor's picks
Trending now
Popular topics