Maths, Physics & Chemistry
Making non-magnetic photons feel a taste for magnetism
Photons are intrinsically chargeless and non-magnetic. Using intrinsic properties of photons and a straightforward structure, we have created “synthetic dimensions” in which such photons behave like electrons, responding to magnetic fields as if they were carrying a charge. The strength of these magnetic field effects can be easily and precisely controlled at will.
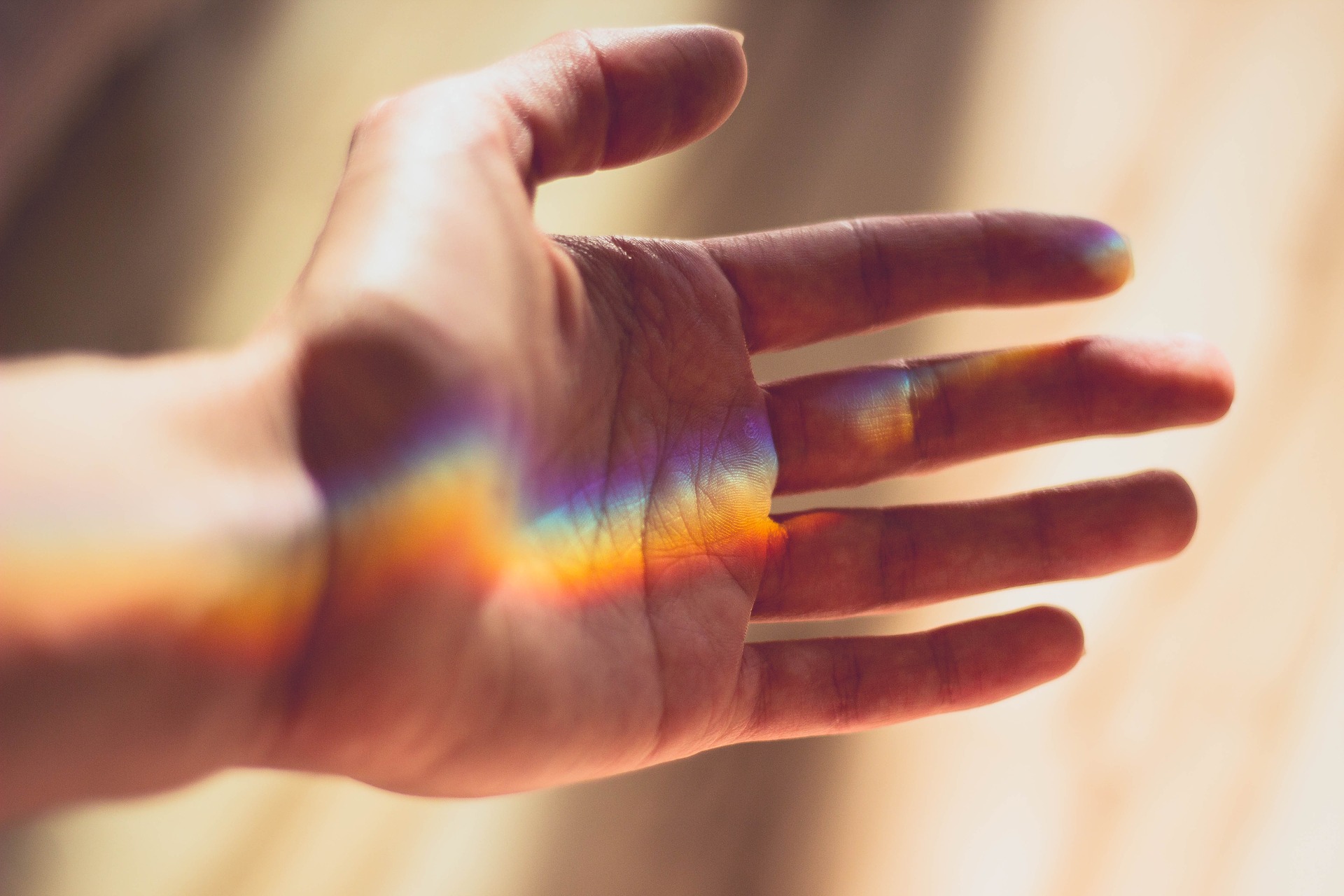
Light consists of chargeless particles called photons. While electrons, being charged, get deflected into curved paths when placed in a magnetic field, the chargeless photons do not feel a real magnetic field the same way. Devising an “artificial” or “fictitious” magnetic field for photons would open up novel ways of controlling them, much like electrons. For example, cathode-ray televisions used magnetic fields to deflect a beam of electrons in a predictable way to paint pictures on a screen.
Achieving such magnetic control of photons has turned out to be quite difficult to date. Previous works have used complicated geometries such as arrays of tens to hundreds of photonic structures to generate artificial magnetic fields for photons. Moreover, the strength of these magnetic fields is fixed by the geometry – unlike a cathode-ray tube where the magnetic field strength can be varied based on the current flowing through a coil. The question naturally arises, is it possible to create magnetic field effects for photons in a much simpler geometry and control its strength at will? Our work shows that this is possible if we can imagine photons' motion beyond the three real directions we are used to – x, y, and z. We term these extra directions of motion as “synthetic dimensions”.
To make this conceptual leap from the three “real” dimensions to synthetic dimensions, we harness internal properties inherent to all photons – frequency and spin. Frequency determines the energy or color of a photon and forms one synthetic dimension. The direction in which a photon goes around in a ring resonator – clockwise or counterclockwise – is another synthetic dimension, “spin”. The ring resonator is essentially an optical fiber looped on itself, such that a photon can go around the loop many times. In real dimensions, one can control whether a photon moves to the right or the left (say in the x-direction). Similarly, our ring resonator can control in synthetic dimensions whether a photon moves up or down in frequency, also imparting a clockwise or counterclockwise spin. Such movement in frequency is achieved with another photonic component called a modulator. This device can change the material’s refractive index at high speeds, making them essential to today’s optical telecommunications networks. Thus, a single ring resonator, which is essentially a zero-dimensional structure, can support two synthetic dimensions due to the multiple frequencies and spin modes.
Having built this single modulated ring with two synthetic dimensions of frequency and spin, we demonstrated a wide variety of complex physical phenomena for photons that are usually associated with electrons in solid-state systems. Most of these phenomena occur when electrons are cooled to very low temperatures close to absolute zero and subjected to a strong magnetic field. Examples of such phenomena include spin-orbit coupling, the quantum Hall effect, and spin-momentum locking. We observe these effects for photons at room temperature in a relatively simple structure consisting of a single modulated ring. As explained before, observing such magnetic field effects for photons has previously proved either impossible or difficult, requiring complex arrays of photonic structures.
In synthetic dimensions, the spin-momentum locking phenomenon means that photons in the ring with a clockwise spin preferentially move up in frequency, whereas counterclockwise-spinning photons move downwards in frequency. In other words, the spin of the photon gets “locked” to the direction of its motion along the frequency dimension, producing “one-way” modes propagating around the two-dimensional array that is reminiscent of the electronic quantum Hall effect.
A significant advantage of synthetic dimensions is the flexibility with which various knobs can be controlled to tune system parameters or even reconfiguring the structure significantly without changing the physical geometry. Such control is unmatched in real spatial dimensions, be it for electrons or for photons. For instance, we showed how the strength of magnetic effects experienced by the photon, such as spin-momentum locking, can be varied by changing the amplitude of the electrical signal applied to the modulator in the ring. Instead, by changing the frequency of the modulator signal, the synthetic dimension structure can be reconfigured.
Our work demonstrates tunable magnetic effects for photons in synthetic dimensions using commercial off-the-shelf components ubiquitous in optical telecommunications. Going forward, miniaturizing the system into tiny chips would enable practical applications such as novel ways to tailor the frequency spectrum of light. Fundamentally, they are promising for quantum simulation of effects beyond three dimensions. For example, one can harness other inherent photonic properties such as polarization or spatial shape to create even more synthetic dimensions.
Original Article:
1. Dutt A, Lin Q, Yuan L, Minkov M, Xiao M, Fan S. A single photonic cavity with two independent physical synthetic dimensions. Science (80- ). 2020;367(6473):59 LP - 64.
Next read: Mitochondria as microlenses in the eye – the evolution of an improved camera sensor by John M. Ball , Wei Li
Edited by:
Massimo Caine , Founder and Director
We thought you might like
Silencing a quantum drum
Feb 27, 2019 in Maths, Physics & Chemistry | 4 min read by David Mason , Massimiliano Rossi , Albert SchliesserMore from Maths, Physics & Chemistry
Testing gravity through the distortion of time
Sep 20, 2024 in Maths, Physics & Chemistry | 3 min read by Sveva CastelloStacking molecular chips in multiple dimensions
Aug 30, 2024 in Maths, Physics & Chemistry | 3 min read by Lucía Gallego , Romain Jamagne , Michel RickhausReversible Anticoagulants: Inspired by Nature, Designed for Safety
Jun 12, 2024 in Maths, Physics & Chemistry | 4 min read by Millicent Dockerill , Nicolas WinssingerDistance-preserving moves always keep a point fixed
May 18, 2024 in Maths, Physics & Chemistry | 4 min read by Shaula FiorelliA resonance triggers chemical reactions between the coldest molecules
Apr 5, 2024 in Maths, Physics & Chemistry | 3 min read by Juliana Park , Wonyl ChoiEditor's picks
Trending now
Popular topics