Maths, Physics & Chemistry
DNA G-Quadruplexes: ‘knot’ that simple!
Known as the ‘molecule of life’, DNA is found in every cell in our body, providing a set of instructions for the function and organization throughout our bodies. DNA’s knot-like structures have been fascinating scientists across diverse disciplines since their presence or absence can ‘turn on’ or ‘turn off’ the instructions in DNA.
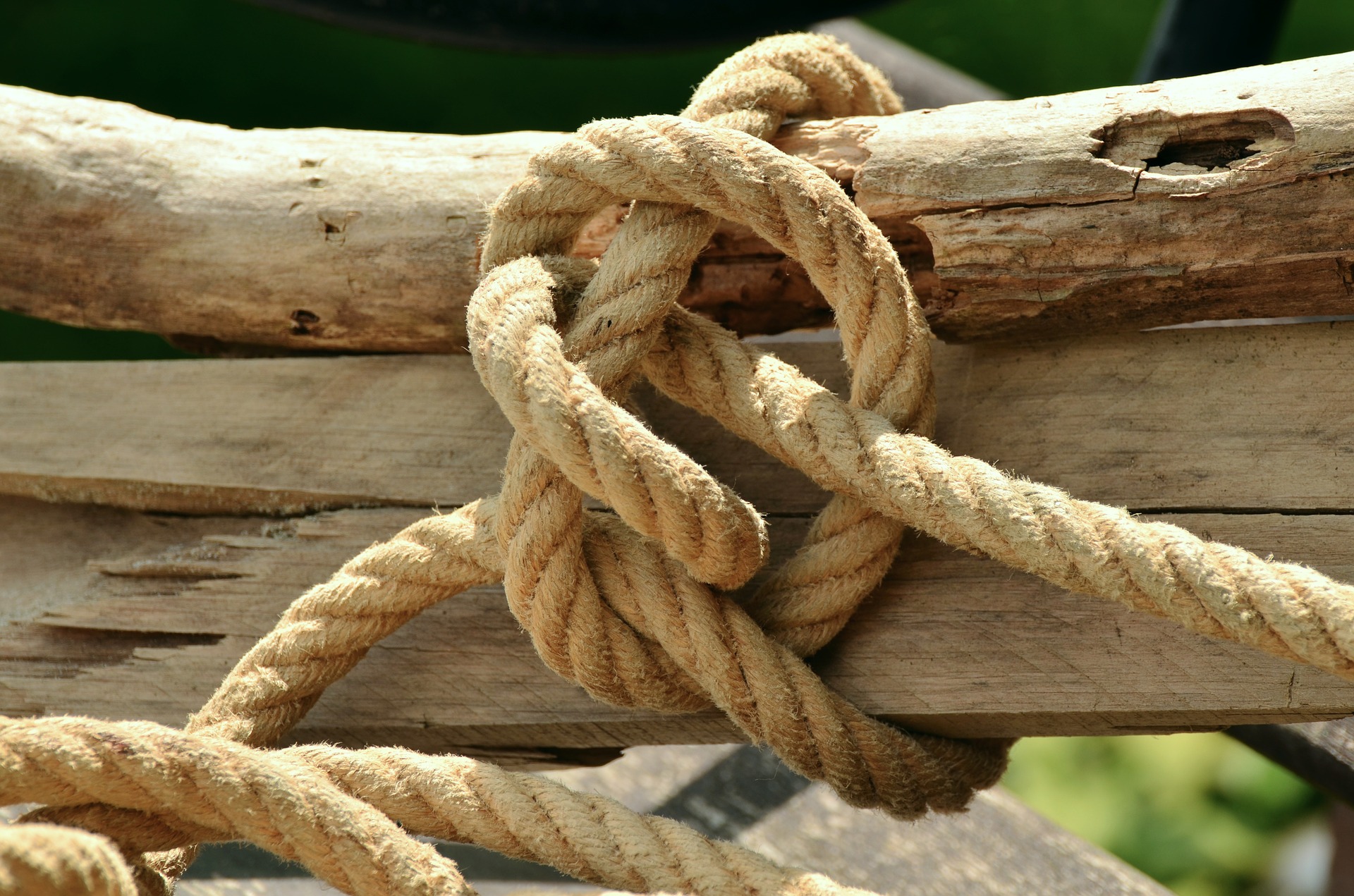
Known as the "molecule of life", DNA is found in every cell in our body, providing a set of instructions for the function and organization throughout our bodies. These instructions are encoded by only four structural variations, abbreviated to A, C, G and T. While the helix shape of DNA is best known to science students around the world, many other shapes of DNA exist as well. Double-helical DNA combines each letter in pairs with one another to create two twisted strands. However, different types of DNA exist, with unique physical characteristics. One of these types, known as "G-quadruplex" DNA, forms knot-like structures out of regions made up mostly of Gs, out of a single strand of DNA. G-quadruplex DNA has been fascinating scientists across diverse disciplines since their presence or absence can "turn on" or "turn off" the instructions in DNA in settings related to diseases. However, little is known about which sections of DNA are able to form G-quadruplexes, the conditions under which they form, or which of several types of "knot" they do form.
We have been studying how to predict and control the formation of G-quadruplexes by observing their formation in laboratory settings. Initially, we focused on defining the range of possible knot types, known as "topologies". Afterward, we focused on how basic structural elements such as the core (made of all Gs) and the parts outside the core, known as "loops", influence the final outcome in G-quadruplex formation. Since many factors can be involved in the final shape of a DNA molecule, we focused on just a few G-quadruplex topologies. Within these shapes, we experimented with different arrangements of letters. In some cases, we changed the number of Gs in the core to see how this affected its size. In other instances, we changed the number or arrangement in loops to determine if this had an impact on which shape of G-quadruplex would form, or if one would form at all.
After designing and preparing over 30 different DNA molecules, we assessed the success or failure of each in forming a G-quadruplex using a technique called Nuclear Magnetic Resonance (NMR) spectroscopy. In its most basic form, this method allowed us to determine if a G-quadruplex had formed by showing the connections between Gs in the core. Once several G-quadruplexes had been identified, we selected the most interesting to investigate in further detail. We used advanced NMR experiments to determine the relationship between each atom in the G-quadruplex, and from this build an experimentally-validated model of the topology formed.
We found new connections between the sequence of letters and their likelihood of making a G-quadruplex. One of the most surprising observations was an interdependent relationship between the size of the core with the number letters in the loops. When the core was larger and more rigid, smaller loops were better. The size of each loop was also influencing the most successful size of other loops. From all of the observations, we were able to define a set of "rules" for describing which features of a short span of DNA are most important for controlling the ability to make a G-quadruplex.
From these results, we were able to conclude that the factors involved in the formation of G-quadruplexes are very complicated indeed. In particular, the core and loops cannot be considered as acting independently from each other. The rules for the formation that were established included specific subsets of shapes formed by the non-core residues, all of which behaved differently from each other. Consequently, the rules defined are only applicable to a strict set of circumstances due to the many inter-relationships identified.
However, this complexity also served to confirm that the biological function of these DNA knots must be highly specific to each set of instructions. The many different shapes possible, and their sensitivity to the arrangement of the letters in the DNA code, demonstrate that different G-quadruplexes are likely to have different functions in the human body, depending on which set of instructions they are attached to. This is promising for other scientists who aim to use G-quadruplexes as a tool to alter the control over harmful or faulty DNA instructions found in some diseases.
Original Article:
S. A. Dvorkin, A. I. Karsisiotis, M. Webba da Silva, Encoding canonical DNA quadruplex structure. Sci Adv 4, eaat3007 (2018)Edited by:
Massimo Caine , Founder and Director
We thought you might like
GMOs are not a human invention: sweet potato is a naturally transgenic food crop
Jul 6, 2015 in Plant Biology | 3 min read by Tina KyndtA new code for a new life
May 26, 2016 in Maths, Physics & Chemistry | 3.5 min read by Jordan CostafrolazAmoebas trap bacteria using nets of DNA: the same mechanism as human immune cells
Jan 27, 2017 in Evolution & Behaviour | 3.5 min read by Lukáš NovákWhat happens to our genes in the twilight of death?
Feb 28, 2017 in Health & Physiology | 3.5 min read by Peter Noble , Alex PozhitkovMore from Maths, Physics & Chemistry
Testing gravity through the distortion of time
Sep 20, 2024 in Maths, Physics & Chemistry | 3 min read by Sveva CastelloStacking molecular chips in multiple dimensions
Aug 30, 2024 in Maths, Physics & Chemistry | 3 min read by Lucía Gallego , Romain Jamagne , Michel RickhausReversible Anticoagulants: Inspired by Nature, Designed for Safety
Jun 12, 2024 in Maths, Physics & Chemistry | 4 min read by Millicent Dockerill , Nicolas WinssingerDistance-preserving moves always keep a point fixed
May 18, 2024 in Maths, Physics & Chemistry | 4 min read by Shaula FiorelliA resonance triggers chemical reactions between the coldest molecules
Apr 5, 2024 in Maths, Physics & Chemistry | 3 min read by Juliana Park , Wonyl ChoiEditor's picks
Trending now
Popular topics